The future may be augmented humans. In any case, companies are working today to design interfaces between the brain and the machine. Neuralink is one of them. This The Conversation article details how these implants work.
Implantable brain-machine electrical interfaces promise major advances, both for understanding how the brain functions and for compensating or replacing functions lost following an accident or neurodegenerative disease: primary vision, motor skills, voice synthesis or digital writing.
While Elon Musk’s Neuralink start-up has just announced that it has placed its first brain implant on a patient, most of these interfaces are still far from being truly operational in the clinic, but they still represent for some the hope of increasing human capacities, with applications that are both sensory (night vision for example) and functional (increase in memory or intellectual capacities for example). Even if many of these applications are still science fiction, such as the transmission of sensation or the increase in our intellectual performance, others do not seem out of reach, such as infrared or ultraviolet vision by example.

Even if ethical questions accompany the development of brain-machine interfaces at Neuralink, the purpose of our article is to explain their technical functioning, their technological issues and the contrast between the hopes they arouse and what they are currently capable of. to realise.
Indeed, current systems are faced with multiple technological and conceptual obstacles. Technical constraints currently limit their use to specific clinical cases, where the risks linked to the insertion of an implant are counterbalanced by the estimation of an immediate or future benefit for patients. We are therefore very far from being able to use these implants in clinical routine and in everyday life, and what is more for recreational applications or even to increase human capacities.


Where is the development of Neuralink and other implants?
For the medical part and the understanding of the brain, the interfaces in development within academic and industrial laboratories already offer interesting perspectives. But few academic tools currently offer a completely implemented solution with as many electrodes and quantity of data as those of the Neuralink interface.
This aims to set up a brain-machine interface that can be implanted in one morning, both for the medical field for paralyzed people, but also to allow everyone to control their smartphone, a video game, or ultimately to increase its human capabilities. For this, it is targeting brain implant technology recording a large number of neurons, which would have no aesthetic impact and would not present any danger.
If Neuralink’s implant proves to work robustly, it could enable progress towards more precise decoding of neuronal activity, the design of clinical neuroprostheses and the understanding of previously inaccessible modes of functioning of the brain. .
Neural implant and neuroprosthesis: how does the Neuralink chip work?
In the literature and current affairs, we find the terms “electrical brain-machine interface”, “neuroprosthesis” or “neural implant” indiscriminately. A “neuroprosthesis” is a type of brain-machine interface that will make it possible to supplement or replace a lost function. Just as the nervous system sends or receives information from its environment, neuroprostheses will capture information from our environment through artificial systems to send it back to the nervous system or capture information from the nervous system to send it back, either towards itself or towards our environment using artificial devices.
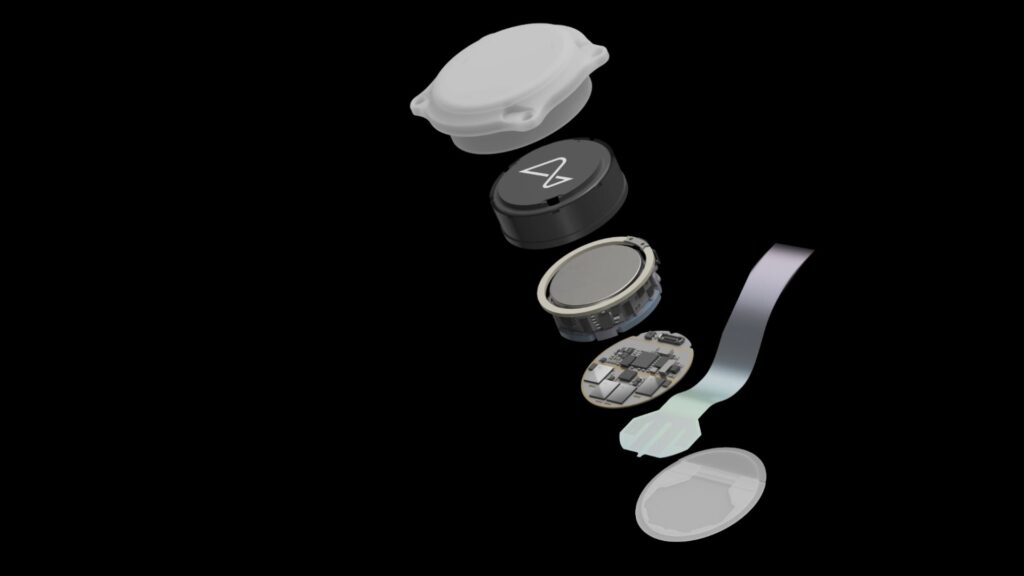
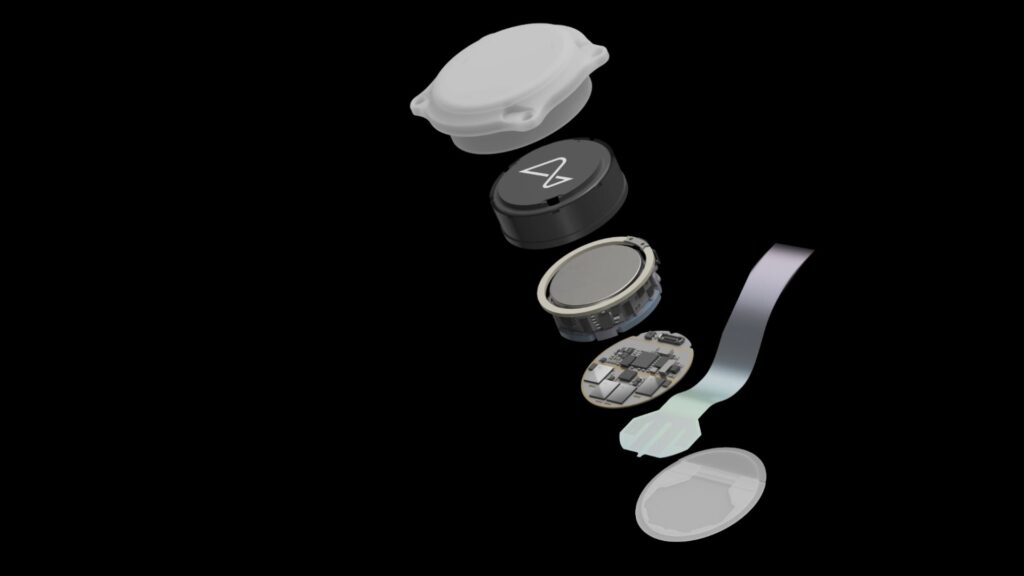
The neuroprosthesis or brain-machine electrical interface is made up of several parts. Going from the neuronal system towards an interface usable by humans (such as a computer screen), the constituents of a neuroprosthesis are as follows: 1) a network of electrodes placed in contact with the neuronal tissue, 2 ) a connection system making it possible to connect the electrodes to an electronic system, 3) a communication system making it possible to send signals to the electrodes or to receive the signals collected by the electrodes, 4) a data recording system, 5) a data processing and decoding system, 6) a system for sending information to one or more effectors, for example, a robotic arm. The implantable part, the “neural implant” strictly speaking, is currently composed of parts 1-2 or 1-2-3.
The limits of current brain-machine interface technologies
The current objective is to have a neuronal implant with a large number of recording or stimulation electrodes, whose effectiveness is maintained over decades. If, despite more than thirty years of research, this objective has not yet been achieved, it is because many major challenges are associated with it, in particular:
- Implantation surgery must be as least traumatic as possible and in particular not damage the micro-blood vessels of the cortex, otherwise it could trigger a significant inflammatory reaction.
- The implant must be as thin as possible, even flexible, so as not to cause too much trauma or a rejection reaction in the brain during its insertion. In addition, ultimately, the protective layer generated by the nervous system can prevent communication between the electrodes and the neurons.
- To record or stimulate as many neurons as possible, it was necessary to develop micro-fabrication methods on flexible micro-devices in order to integrate as many electrodes as possible in a very small space. Current electrodes can reach sizes of around 5 to 10 micrometers.
- Many new electrode materials have been developed to detect or stimulate the very weak electric fields generated by neurons, which was not possible with conventional metals such as platinum. Today, the performance of electrodes has been greatly improved, in particular thanks to the introduction of porous materials.
- The implant must maintain the integrity of its electrical performance over time, but current flexible technologies are sensitive to water in the long term, which affects the lifespan of the implants. This point is one of the major technological obstacles.
- In order to be able to move normally outside a laboratory or hospital, the implants must be able to communicate and supply each other with energy, without wires. But current technologies for radiofrequency transmission of signals, when there are numerous electrodes, cause a local rise in temperature which is harmful to neuronal tissues – another major technological barrier.
How to make these brain-machine interfaces a reality?
To try to resolve these problems, the company Neuralink has, for example, designed a network of electrodes to stimulate or record neuronal activity, distributed over several flexible polymer filaments which carry microelectrodes. The materials used are biocompatible and layers of silicon carbide to ensure the electronic integrity of the implants appear to be under study (a concept from research laboratories at the University of Berkeley and also under development in France as part of the SiCNeural project funded by the ANR). Finally, each filament is connected to an electronic chip that is used to record neuronal activity or generate electrical pulses for stimulation.
In addition, the company is developing an autonomous robot capable of carrying out all stages of implantation surgery, from trepanation to insertion of implants.
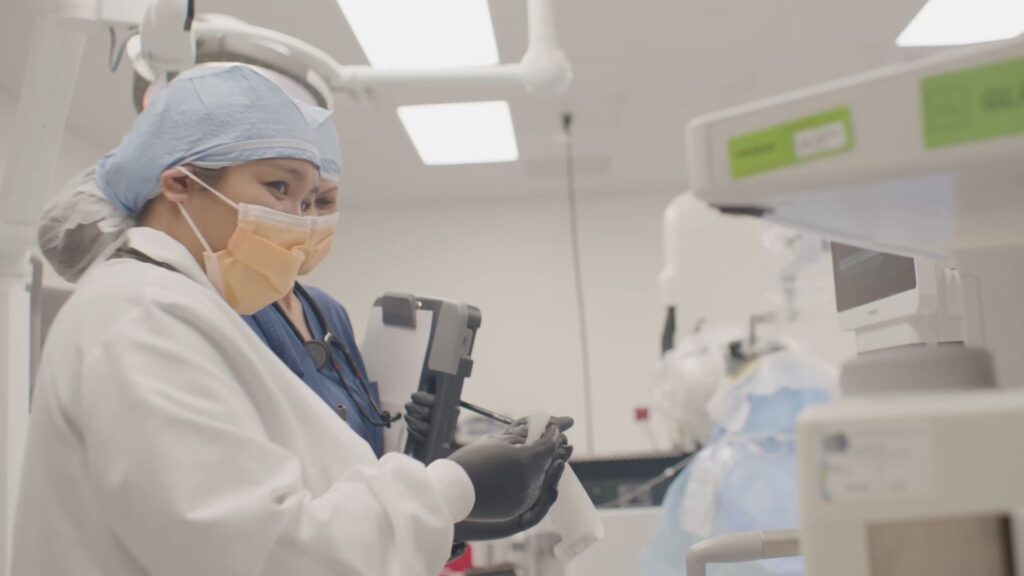
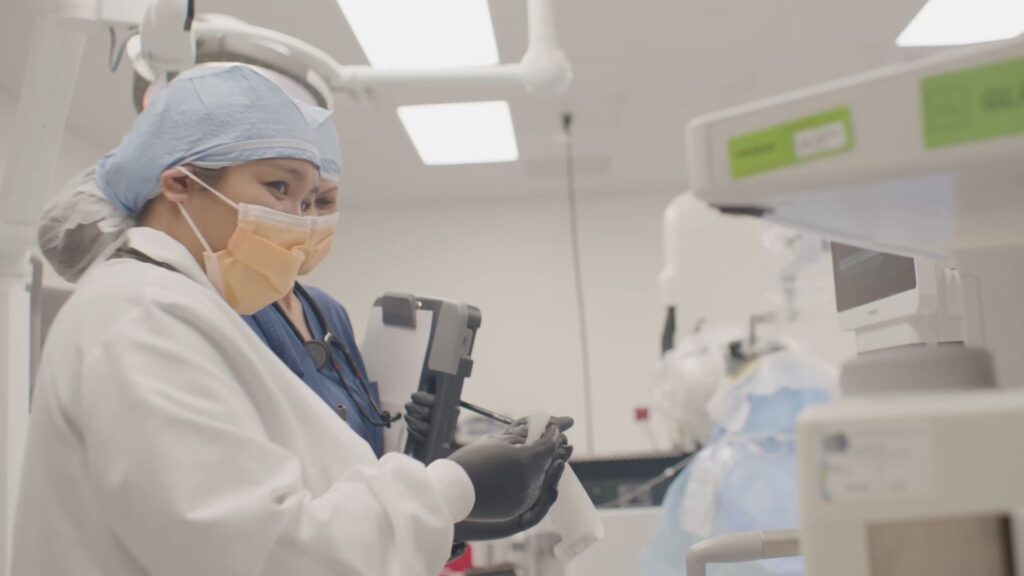
The insertion of flexible implants into the brain is in fact not simple and several strategies have been developed by different laboratories, such as the temporary stiffening of the implant using a resorbable polymer, the use of a rigid guide or a robotic “sewing machine” approach, also developed at Berkeley, which threads a needle through a hole at the end of the flexible implant to push the implant into the brain and then remove only the needle. This last method is taken up by Neuralink, which combines it with a system of cameras identifying areas of the surface of the cortex with no or little vascularity where implants can be inserted while limiting microbleeding.
Avoid overheating during data analysis and transmission
As for the problem of local heating due to the analysis and wireless transmission of data, two technologies had until then been applied to humans.
The first is that of the company BlackRock Neurotech, which deports the processing and sending circuits of signals above the cranium. This generates aesthetic problems but also risks of infections because of the wires running from the skin to the brain.
The second technology is that of the CLINATEC laboratory at CEA Grenoble, which only collects signals that do not require high digitization precision and only records information on a maximum of 64 electrodes simultaneously. This laboratory has thus produced the first wireless neural implant with so many channels, and completely integrated under the skin. It is inserted to replace part of the skull bone. Neuralink, for its part, offers a smaller chip, also inserted into the skull bone, processing more than 1000 channels, but only sending certain characteristics of the neural signals, deemed important thanks to on-board algorithms.
Concerning the lifespan of the implants, we will still have to wait a little to see if the strategy is effective and allows us to have a stable interface over several years. Once this limit is exceeded, it will certainly be necessary to tackle the collection of an even greater number of signals. At present, we can estimate that Neuralink technology can record up to around 3,000 neurons with its 1,024 electrodes: this is impressive from a state-of-the-art point of view, but very far from be sufficient to understand the immensity of brain signals.
Conceptually, despite very good miniaturization, it will be very difficult to achieve the recording of millions of individual neurons with this technology without the implant and the associated connectivity taking up too much space in the brain. Other concepts may need to be imagined to go beyond these limitations.


Clément Hébert, Researcher in neural implants, neuroprostheses, Inserm U1216 Grenoble Institute of Neurosciences, Grenoble Alpes University (UGA) and Blaise Yvert, Research Director at Inserm, head of the Neurotechnologies and Network Dynamics team, Inserm
This article is republished from The Conversation under a Creative Commons license. Read the original article.
Subscribe to Numerama on Google News so you don’t miss any news!